buy cheap cialis super active by mail pharmacy

The current pandemic of coronavirus disease 2019 (COVID-19), caused by severe acute respiratory syndrome coronavirus 2 (SARS-CoV-2), has triggered an intensive hunt for effective new and repurposed antivirals and antibodies, buy generic coreg overnight shipping no prescription for prevention and treatment of the infection.
A new preprint released on the bioRxiv* server addresses an urgent need – namely, a therapeutic antibody directed specifically against a key SARS-CoV-2 antigen which is highly conserved among similar viruses, and is not easily bypassed by viral escape mutations.
.jpg)
Spike-targeting neutralizing antibodies
SARS-CoV-2 possesses multiple spikes on its surface, which mediate its attachment to the target host cell receptor, the angiotensin-converting enzyme 2 (ACE2), and viral entry into the cell. The spike-receptor interactions mostly take place at the receptor-binding domain (RBD) on the S1 subunit of the spike.
The extent of protection from infection in the case of SARS-CoV-2 is probably linked to the neutralizing antibody titer. Those with the most potent neutralizing ability are directed against the RBD and are found to predominate in polyclonal sera from convalescent COVID-19 patients and in COVID-19 vaccine recipients, as well as in therapeutic antibodies.
The recently emerged South African (SA) and Brazil variants show varying resistance levels to vaccine-induced neutralizing antibodies. These are characterized by mutations affecting the RBD, which impact its binding to such antibodies.
Study aims
The current study seeks to explore both the potential existence of such antibodies, as well as to find antibodies that have broad activity against SARS-related coronaviruses (Sarbecoviruses). These would be essential, given the future potential for outbreaks of other Sarbecoviruses.
The researchers in this study worked on a previously reported antibody S309, which triggers potent effector activity and has neutralizing activity against currently circulating SARS-CoV-2 isolates as well as SARS-CoV. This was developed as the therapeutic antibody VIR-7831, which shows clinical efficacy in treating COVID-19.
The attempt is to better understand how the RBD antibody-binding sites or epitopes are related to the potency of neutralizing antibodies, resistance to viral evasion mutations, and the cross-reactivity of such antibodies to other Sarbecoviruses.
The researchers made use of a panel of different antibodies using binding assays, deep mutational scanning, positive selection of viral escape, and the biochemical and structural characteristics of such antibodies. Six of these antibodies were reported for the first time in this study.
The antibodies studied here bound a variety of epitopes, including those that overlap the receptor-binding motif (RBM) in the RBD, and those within the RBM itself, and show a range of RBD-binding affinities and neutralizing potencies against both wildtype and pseudoviruses particles expressing the SARS-CoV-2 spike antigen.
Effect of size of escape mutation
There are many different sites of mutational escape, depending on the antibody. With narrow functional epitopes (a sequence that is crucial to antibody binding), a single or a few key mutations could abolish RBD binding. One such antibody is S309. Others, such as S2H13, have broad functional epitopes.
Mapping escape mutations
In order to predict the effects of such mutations, the investigators had previously assessed the effect of all RBD mutations on the binding affinity to the ACE2 receptor, and on the higher structure of the protein – which determines its function.
These effects were combined to produce a computed measure of how easy it is to escape neutralization by each antibody – an escape mutation map. This, in turn, reflects viral tolerance for such functional mutations.
The study also looked into the sensitivity of each antibody to mutations reported in the GISAID (Global Initiative for Sharing All Influenza Data) database up to March 4, 2021.
The researchers found that the impact of natural mutations in the viral RBD was much greater for some antibodies than for others. These include mutations found in fast-spreading viral strains, called the variants of concern (VOCs).
They also found that of the four antibodies that bind to the core RBD, cross-reactivity and tight binding were observed with SARS-CoV and other ACE2-binding Sarbecoviruses with over 70% amino acid identity in the RBD.
In addition, S304 and S2H97 showed the ability to cross-bind other more different non-ACE2-binding RBDs with only 64% identity to the current virus.
“S2H97 [is] the broadest pan-sarbecovirus RBD antibody described to date.” Pseudovirus assays confirmed that S2H97 neutralizes a variety of different spike variants from both SARS-CoV-2- and SARS-CoV.
Antibodies that bind only RBM-restricted epitopes show much lower cross-binding, involving only the RBD of the SARS-CoV-2 and the pangolin CoV.
A unique finding was that S2E12 binds to the RBD of all SARS-CoV-2 variants and to the pangolin CoV, which has only 86% identity with the former. This suggests that some functional epitopes tolerate more substitutions than others within the constantly evolving RBM.
Structural basis of neutralization potency
The earlier escape mutation mapping was used to understand how similarly binding escape mutations look in two dimensions. It shows how epitopes move across the RBM in a smooth progression, beginning from that binding to the Lilly antibody LY-CoV016 through S2X58 to that produced by Regeneron, REGN10987.
The 2-D projection also shows which antibodies bind to unique parts of the RBD. For instance, S2H97 engages with the side of the core RBD, below the receptor-binding ridge, adjacent to the N-terminal domain (NTD) of the neighboring protomer of the trimeric spike protein, in the closed configuration.
Connecting the in vitro neutralization potency of various antibodies, their binding spectrum and immune evasion properties with this projection allowed an exploration of their structural basis.

Antibodies with the greatest neutralizing ability had the narrowest functional epitopes, binding within the RBM epitopes.
Conversely, those binding to the core RBD epitopes showed lower levels of neutralization. Of course, neutralization is not the only mechanism of protection against infection for RBD-binding antibodies.
Antibodies with broad sarbecovirus binding universally target the core RBD, illustrating a general tradeoff between breadth of sarbecovirus binding and potency of SARS-CoV-2 neutralization.”
Location and immune evasion
The number of RBD escape mutants within the functional epitope of each antibody (its “size”) is not closely related to its location. Instead, narrower functional epitopes, with fewer escape mutations, have higher binding affinity between the Fab (antigen-binding fragment) and the RBD.
However, the location does affect the link between the size of the functional epitope and its potential for immune escape. The S2E12 and the S2X58 antibodies have similar functional epitope breadth, unlike the narrower functional epitope of S2D106.
S2E12, however, binds to crucial ACE2-contacting amino acids, where mutations would impair the secondary folding of the RBD, abolishing its function. Few such escape mutations have been observed.
S2E12 can bind multiple Sarbecoviruses showing significant identity to SARS-CoV-2, including the bat RaTG13 and pangolin CoVs. Indeed, a broader range of binding among core RBD antibodies indicates high affinity binding and low escape breadth, since they bind to sites with functional constraints.
Conversely, S2X58 and S2D106 bind to epitopes that can vary across different SARS-CoV-2 variants, indicating functional tolerance.
As expected, of the seven antibodies tested for positive selection of the escape mutants, the use of S2X58 led to the emergence of viral variants with multiple scattered mutations. S2D106 allowed only mutations at two sites.
Escape mutations selected by these antibodies include L452R and E484K, as found in the VOCs.
However, S2E12 selects for mutations at few sites associated with low ACE2 binding affinity. These mutations, being functionally constrained as well as under less selection pressure by natural antibodies in polyclonal sera, are not found in VOCs.
Structural basis for escape mutations
The reason for this difference is structural. S2E12 binding to the RBD core causes packing of RBD F486 and N487 residues within a buried hydrophobic cavity, at the junction of antibody heavy and light chains. The first residue makes contact with the ACE2 receptor, while the second forms polar contacts with the antibody backbone.
In contrast, S2E12 binding sites such as E484 and S477, at the periphery of the ACE2-RBD interface, have more variation in sequence, being less functionally constrained. This explains why S2E12 binds with high affinity to common mutants at these sites.
It also explains its binding to RaTG13 and pangolin CoV, with the F486L mutation that allows similar packing in the same hydrophobic cavity.
With S2D106 binding, the antibody is tethered by a buried polar contact between RBD E484 and antibody R96 residues, and by nonpolar contacts between the RBD F490 and the heavy chain complementarity domain region 2 (CDR2) of the antibody.
With no indispensable role in binding, the E484 and F490 residues can easily harbor escape mutations while retaining their function.
This comparison highlights how a subtle change in the focus of the RBD: antibody interaction impacts the robustness of each antibody to viral escape, including among natural SARS-CoV-2 mutants.”
Structural impact for antibody breadth
The study also shows that S2H97 is so broad in its binding across Sarbecoviruses because it binds to a hitherto unrecognized highly conserved site V. During this binding, the heavy chain CDR3 is packed into a crevice of the RBD occurring at the center of the epitope.
Additionally, all three heavy chain CDR3 loops and the light chain CDR2 make crucial polar interactions with the RBD, making this a functionally constrained binding surface.
Mutations here impair RBD folding, especially with quaternary NTD packing in the closed state of the trimeric spike. Moreover, this binding surface is open only with a widely opened RBD. This explains the scarcity of antibodies to this site and the low neutralization potency of S2H97, despite its high affinity.
S309 binding binds to a small area of the RBD and thus does not permit significant diversity of structure. The escape mutations here involve E340 and P337 residues, with electrostatic and van der Waals interactions with CDRH3.
The former residue is key to antibody contact. Mutations at the P337 residue allow escape by steric hindrance or by loss of stability of E340.
The narrow binding epitope means that S309 neutralizes SARS-CoV-2 mutants in current circulation and also binds RBDs of other Sarbecoviruses that have a conserved E340, despite having eight different residues within the binding area of this antibody.
S2X35 has a large binding interface, but escape here is not because of changes in the side chains that are necessary for antibody binding. Instead, the G504 residue, when mutated, creates steric hindrances with D405 and the antibody’s light chain. D405 mutation entails very drastic changes in the sequence. This low potential for escape indicates no single RBD side chain is responsible for its binding to the antibody, which in turn allows broad sarbecovirus binding.
What are the implications?
Rather than a single-minded quest for increased potency of neutralization, important as this aspect is, these results indicate that “screening antibodies for high-affinity binding and sarbecovirus breadth identifies antibodies that are robust to ongoing SARS-CoV-2 evolution.”
As VOCs emerge, vaccine-elicited antibody resistance is a major concern. Most VOC mutations are in the RBM, including E484, K417 and L452. These prevent binding by polyclonal serum and certain therapeutic monoclonal antibodies. Five antibodies in this panel, targeting the RBM, could be evaded by mutations at position 484.
The use of escape maps could help identify antibodies that resist such immune escape and the selection of therapeutic antibodies against the variants in circulation at the time in that locality.
The future may include newly dominant mutations, including some in the RBM that prevents antibody binding. Thus, antibodies that recognize broad epitopes should be focused on, as well as epitopes that are highly conserved, including residue-level differences among the various epitopes. This will help identify and implement better interventions that resist such immune escape both in the current pandemic and in the future.
*Important Notice
bioRxiv publishes preliminary scientific reports that are not peer-reviewed and, therefore, should not be regarded as conclusive, guide clinical practice/health-related behavior, or treated as established information.
- Starr, T. N. et al. (2021). Antibodies to the SARS-CoV-2 receptor-binding domain that maximize breadth and resistance to viral escape. bioRxiv preprint. doi: https://doi.org/10.1101/2021.04.06.438709. https://www.biorxiv.org/content/10.1101/2021.04.06.438709v1
Posted in: Medical Science News | Medical Research News | Disease/Infection News | Healthcare News
Tags: ACE2, Amino Acid, Angiotensin, Angiotensin-Converting Enzyme 2, Antibodies, Antibody, Antigen, binding affinity, Cell, Coronavirus, Coronavirus Disease COVID-19, Efficacy, Enzyme, Evolution, Genetic, Glycosylation, in vitro, Influenza, Mutation, Pandemic, Protein, Pseudovirus, Receptor, Respiratory, SARS, SARS-CoV-2, Severe Acute Respiratory, Severe Acute Respiratory Syndrome, Spike Protein, Syndrome, Vaccine, Virus
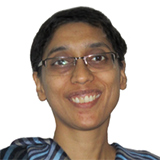
Written by
Dr. Liji Thomas
Dr. Liji Thomas is an OB-GYN, who graduated from the Government Medical College, University of Calicut, Kerala, in 2001. Liji practiced as a full-time consultant in obstetrics/gynecology in a private hospital for a few years following her graduation. She has counseled hundreds of patients facing issues from pregnancy-related problems and infertility, and has been in charge of over 2,000 deliveries, striving always to achieve a normal delivery rather than operative.
Source: Read Full Article